Tissue-Engineered Implant Requires Less Invasive Surgery
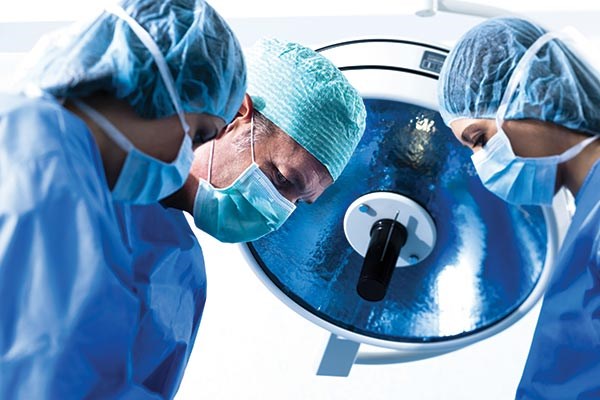
11/02/2016
By Edwin L. Aguirre
The human heart is an engineering marvel, an efficient machine that beats an average of between 60 and 100 times per minute in healthy adults. That’s equivalent to between 86,000 and 144,000 heartbeats each day.
An integral part of the heart that enables it to pump blood efficiently and keep it circulating in the right direction is the valves. Made of strong, thin flaps of tissue called leaflets, the heart’s four valves act as one-way inlets and outlets, opening and closing the heart’s four chambers with each beat.
Age, birth defects, infections, rheumatic fever or other conditions can cause one or more of the valves to not open fully or allow blood to “leak” back into the heart chamber, impeding its ability to pump blood and making the heart work harder. If not treated, severe valve disease can cause premature heart failure, blood clots, stroke or cardiac arrest. Currently, no medicines are available to cure heart valve disease. Eventually, the faulty valves may need to be repaired or replaced through surgery.
“Traditional artificial valve replacements currently used by doctors can harden or wear out over time,” says mechanical engineering Asst. Prof. Scott Stapleton, a researcher at the university’s Advanced Composite Materials and Textile Research Laboratory. “And open-heart surgeries are very invasive and carry high risk of death.”
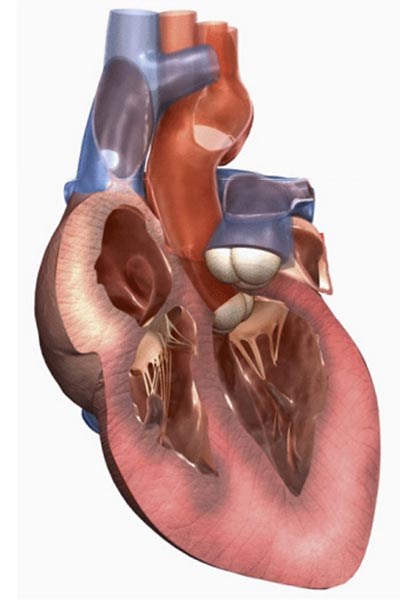
“Tissue-engineered means the valve is grown from the patient’s own cells to create a living and growing organ,” explains Stapleton. “This also means there is less chance of the body rejecting the implanted valve.”
Unlike artificial mechanical valves that can cause potentially lethal blood clots to form, patients with tissue-engineered valves are not required to take blood-thinning medicines for the rest of their lives, which is especially beneficial to pediatric patients.
Another upside for young patients is that the valve will continue to grow with them so they would probably not need follow-up surgery to replace the valve after about 10 to 15 years. This lessens the emotional, physical and financial stress on patients and their families.
Reinforcing the Valve with Textile Fibers
According to Stapleton, most tissue-engineered valves under study today are well suited for replacing the pulmonary valve, which allows oxygen-poor blood to be pumped from the heart to the lungs. But they are currently not strong enough to withstand the high pressures found on the aortic side of the heart, where oxygen-rich blood from the lungs is pumped forcefully from the heart out to the rest of the body. To strengthen the tissue-engineered valve’s structure, Stapleton and his colleagues have reinforced the valve with a scaffold made of polyester (polyethylene terephthalate, or PET) textile.
“We have to make sure that there is enough reinforcement to improve the valve’s strength and durability, but not so much that the valve becomes too stiff and cannot open and close fast enough. If the valve is too soft, it will tear under pressure,” notes Stapleton. “Therefore, the textile-reinforced valves must be carefully engineered.”
To aid the team in designing the textile reinforcement, a computer model is being created that will look at the tissue, the textile and the entire valve to predict its strength and closing speed.
“The growth and development of the cells around the textile is particularly difficult to capture, and it is not known what effect the textile will have on the cells. Once the model is created and shown to work correctly, it will be used to compare different textile designs to make a strong, functional aortic heart-valve prosthetic,” says Stapleton.
He adds: “My work involves mainly the numerical modeling of the valve to account for tissue growth and adaptation to load during the conditioning process, as well as modeling the effects of the textile reinforcement on the valve’s mechanical performance. My primary goal is to gain insights into how the tissue-engineered valves work and how to improve them. My colleagues at Aachen University are the ones conducting the actual tissue engineering and clinical trials.”
The team’s findings were reported last year in the journal, Advanced Modeling and Simulation in Engineering Sciences.