Innovative Approach Could Lead to New Treatments for Life-threatening Infections
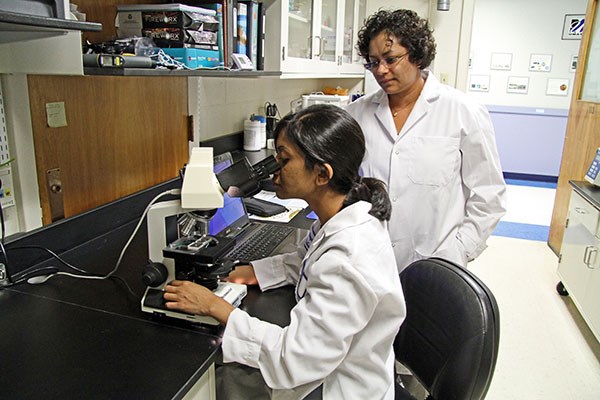
10/10/2019
By Katharine Webster
Four years ago, Assoc. Prof. Bridgette Budhlall’s father died of MRSA, an antibiotic-resistant strain of the staph bacterium that infected him while he was hospitalized for complications from diabetes.
That set the plastics engineering professor to thinking about how to design polymers to treat infections caused by “superbugs” like MRSA – bacteria that have evolved to resist antibiotics. Hospital-acquired infections, most of them caused by such superbugs, are a leading cause of death around the world and account for an estimated 100,000 deaths each year in U.S. hospitals alone, according to the U.S. Centers for Disease Control and Prevention.
Budhlall says that other researchers had experimented with making biopolymers to attack such superbugs. Those biopolymers all attempted to mimic human antimicrobial peptides, proteins that bind to and kill invasive bacteria. All of them had a water-repellent (hydrophobic) component and a water-loving (hydrophilic) component, just like antimicrobial peptides. But some proved toxic to many kinds of cells, not just the infection-causing bacteria.
Then, a “very creative and innovative” Ph.D. student working in Budhlall’s lab, Amit Garle, found an article in the journal Nature Materials that opened up new possibilities for their research. The article suggested that polyethylene glycol (PEG), a water-soluble polymer that has low toxicity and is commonly prescribed as a laxative, could be deployed to damage cell membranes structurally.
Instead of mimicking human antimicrobial peptides, Budhlall and Garle decided to design a polymer using PEG that would attack the cell walls of bacteria like a toothbrush scrubbing a grape.
“Chemistry is not the only thing that matters. Physics, governing the conformational stress exerted by the molecule, is key to rupturing membranes,” Budhlall says.
Budhlall and Garle decided to target gram-negative bacteria, which cause plague, cholera, typhoid fever, gonorrhea, meningitis, severe diarrhea and many other diseases. They also cause four of the most common hospital-acquired infections: pneumonia, urinary tract infections from catheters, surgical site infections and septicemia.
There are far fewer effective antibiotics for gram-negative bacteria than for gram-positive bacteria, because gram-negative cell membranes have a complex structure and a different outer layer that not only makes them harder to kill, but carries toxic proteins that can cause bloodstream infections.
To rupture that outer layer, the scientists created a “bottle-brush” polymer with a positively charged “backbone” and lots of long, wavy “bristles,” or side-chains, made out of PEG, then tested it on substances designed to mimic the cell membranes for E. coli – a gram-negative bacterium – and red blood cells.
Their polymer selectively damaged the bacteria-like membranes, but not the red blood cell-type membranes. Budhlall’s and Garle’s hope is that, if it works in the same way with live cells, the damage to the gram-negative bacterial cell walls would cause the cells to weaken and die without harming the patient’s own cells. Their results were published in Langmuir, a publication of the American Chemical Society and the leading journal in the field.
“I’m really proud that we came up with a polymer, showed its efficacy and proposed a mechanism that explains how it works,” Budhlall says. “Our scientific discovery is that you don’t need molecules that mimic antimicrobial peptides.”
The new polymer’s “backbone” has extra positive ions on the outside, so it is attracted to the negative ions on the outside of gram-negative bacteria. As that attraction creates a tight bond between the backbone and the cell wall, the PEG side-chains on the polymer, the “bristles,” are forced to bend out of the way.
Budhlall demonstrates with an actual bottle-brush used for washing out test tubes. It consists of a twisted wire spine that radiates fine bristles. She spreads the bristles apart forcefully so that she can lay her finger, representing the cell wall, lengthwise along the wire spine of the brush.
Spreading the bristles creates stress, she explains: They want to return to their original configuration, so they press up against and start pricking her finger. The PEG side-chains on the bottle-brush polymer do the same thing. “They essentially snap back and force their way through the membrane,” she says, causing leakage that would eventually lead real cells to die.
Budhlall did the research without outside funding. The next step for her and Garle, now a postdoctoral fellow at the Mayo Clinic in Rochester, Minn., is to apply for a grant that will allow them to test their polymer on real E. coli and red blood cells.
Their discovery opens up a new pathway for research into polymers that can selectively destroy gram-negative bacteria. And, because the polymer is water-soluble, it could be easier to formulate as an intravenous or oral medication than other polymers that are partly hydrophobic. “This expands the shelf of molecules that can potentially be used to treat life-threatening infections,” she says.