Physicist Archana Kamal Is Leading UML’s Efforts to Advance Quantum Computing
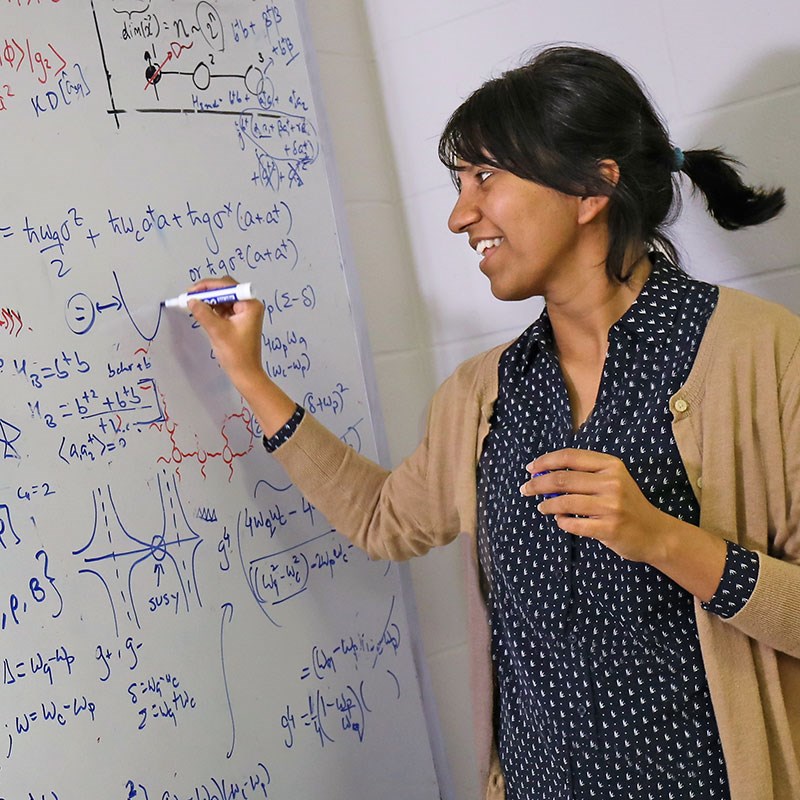
04/25/2022
By Michael Blanding
There’s another world beneath our fingertips. You can’t see it — it’s far too tiny for that. But beneath the level of cells and molecules, beneath even the level of atoms, the quantum realm obeys a completely different set of laws. Principles we take for granted in the visible realm of classical physics, such as the idea that we can know where an object is at any moment in time, completely break down in quantum physics.
“Quantum information tells us that the way nature processes information is actually very different from the way we think of information processing,” says Archana Kamal, assistant professor of physics. “You can’t know where something is at a given time with absolute precision, because nature doesn’t process information like that. It’s not deterministic — it only lets you do just enough to predict something with a very high probability.”
The discovery of quantum mechanics nearly a century ago was one of the most disturbing and revolutionary advances in science. “It may be a humbling experience for human beings to know that they are not the masters of the universe who can control everything,” Kamal says. “On the other hand, it allows you to have a completely new outlook on the world.”
Recently, quantum physicists and computer scientists have begun to harness that outlook, simulating quantum mechanics with classical materials to create quantum computers, which theoretically have power exponentially greater than even the most advanced supercomputer. These quantum processing systems use quantum’s uncertainty to its advantage: Instead of laboriously calculating the states of bits and bytes of information at a very moment, they can use probability to estimate them, shortcutting vast swaths of calculations and allowing quantum computers to operate at lightning-fast speeds.
The resulting computers could precisely optimize the most complicated transportation system, create new vaccines with machine learning in record time or discover vastly more efficient new materials for creating solar power. Companies including Google, Microsoft, Intel and IBM — as well as venture-funded startups — are all racing to capitalize on the technology. The White House, meanwhile, held a summit on quantum computing last fall, and the Biden administration is investing $180 million in R&D to develop advanced computing infrastructure.
Reshaping the Future, Shedding Light on the Past
Quantum computing could not only reshape the future, but it could also help us understand the past: The ability to simulate the quantum realm could help us unlock the nature of how the universe was created, and allow us to create exotic quantum matter beyond basic elements that appear on the Periodic Table. “It’s not just about computational power, it is about understanding the most fundamental processes that underlie everything we see — or don ’t see — around us,” says Kamal.
Of course, nothing comes without a catch, and beyond the hype, nature hasn’t been willing to give up its secrets so easily.
Despite the incredible progress that has been made with quantum computing over the past decade, scientists have been faced with a fundamental problem: Simulating quantum states with materials traditionally used in computing is inherently unnatural. While researchers have been able to put a handful of quantum bits — or qubits — into quantum states long enough to perform some impressive calculations, they tend to fall out of those states easily, and noise and errors prevent them from reaching anywhere near their full potential.
That’s where Kamal comes in. As a theoretical physicist, she has come up with new techniques to harness the power of quantum information. Her unique methods for getting quantum and traditional computers to talk with each other earned her a spot on MIT Technology Review’s prestigious list of “35 Innovators Under 35.” And her research group QUEST (Quantum Engineering Science and Technology) has scored some impressive government grants — totaling almost $5 million in all — to pursue a new form of error correction that could finally keep qubits in line long enough to work. See article on another grant related to the evolution of the universe.
Quantum computers don’t look anything like the kind of hardware we are used to. In their most common form, they consist of a solid-state chip that at extremely low temperatures (say, below -270 degrees Celsius/-454 degrees Fahrenheit) becomes superconducting and begins to take on quantum characteristics. Among those qualities is the ability to stay in “superposition,” the state of uncertainty between a 1 and 0 that gives quantum computing its power.
Additionally, qubits can enter a state of “entanglement” with another qubit, so it’s impossible to describe the state of one without the other. The more qubits that are entangled, the stronger the quantum computer’s power. In 2019, Google released a computer with 54 qubits that the company claimed passed the point of “quantum supremacy” — solving a mathematics problem that would be impossible on a classical computer. The current leader in the quantum computing race, IBM, just released a new computer with 127 qubits. At 300 qubits, a quantum computer could theoretically perform more calculations per second than the number of known atoms in the universe.
Will Quantum Live up to the Hype?
The looming question is whether these computers will overcome the challenges and live up to the hype. Their tendency to fall out of quantum state, become disentangled and produce errors significantly slows down their speed and efficacy. Another challenge has been how to route and amplify the weak quantum signal to be read by a comparatively big classical computer. In the past, scientists have solved that problem by using powerful magnetic devices that break bilateral symmetry.
“But you can’t integrate such magnetic devices on the same chip as superconducting qubits being used by companies such as IBM and Google,” Kamal says. “In fact, it leads to the introduction of a very complicated magnetic shielding apparatus to protect superconducting qubits from magnetic fields, which all leads to a prohibitively large measurement and control chain for even a few-qubit system. Essentially, such conventional ways of symmetry breaking turn out to be a nonstarter for scalable quantum processors.”
All that complicated machinery, says Kamal, creates a lot of noise that interferes with the signal being transmitted — like a loudspeaker creating static. “The system loses its ‘quantumness’ in the presence of noise, so you cannot do any kind of information processing,” says Kamal, who sought to produce a more elegant solution to boost the signal on the chip itself, without the need for such complicated machinery.
Originally from India, Kamal studied theoretical physics at the Indian Institute of Technology in Delhi and earned a master’s degree and Ph.D. in physics at Yale. It was there that she encountered quantum information processing, which at the time had a different name. “We used to call it mesophysics, because the systems were large enough that you could control them, but small enough that they were still quantum,” she says. The potential of the new field spurred her curiosity: “It defies boundaries by construction and gives you a lot of breakthroughs to explore.”
At Yale, Kamal focused on experiments using quantum circuits. As a postdoc at MIT, however, she gravitated to the theoretical, giving her the background to tackle problems from both an abstract and concrete perspective. “I just found myself doing more and more theory, but I maintained very close connections with experimental groups,” she says. The combination of perspectives allowed her to conceive of a new way to boost the quantum signal without using bulky magnets — using light instead.
Cutting Down the Noise
Unlike electricity, light doesn’t flow in a particular direction — it travels in both directions at once. “That’s a big problem for us, since any loss of information leads to decoherence,” says Kamal. The challenge was making sure there is a unidirectional flow, since the goal is for the signal to have a clean one-way route from quantum computer to classical computer. Kamal came up with an elegant solution, setting up an interference phenomenon that is not only direction-dependent but also simultaneously amplifies the number of photons flowing in the chosen direction, making it easier to read quantum information. Essentially, such directional amplification of photons allows a classical user to access information in the quantum realm with minimum corruption or noise. Her proposed solution has quickly become a standard functionality used by leading quantum computing companies and research labs.
Since joining UMass Lowell’s faculty in 2016, Kamal has won a series of government grants to investigate new techniques to address decoherence, which is also known as dissipation. The phenomenon refers to the tendency for qubits to fall out of entanglement, thus limiting their computing power and introducing errors into calculation. “Entanglement is a very fragile thing, because the information isn’t contained in either place, but in the relationship between the two,” she says. “If anything happens to either of them, the information goes away.”
Quantum computer scientists typically solve this problem by implementing error correction codes that detect and discard errors within the quantum logic gates of the circuit. However, such techniques slow down speed and power, especially as the number of qubits increase. With the help of her QUEST group, Kamal is exploring a very different approach to the problem — asking “What if the qubits never decohered in the first place?” In 2019, the U.S. Department of Energy awarded her a $1.5 million grant to pursue the question, supplemented by an additional $1.85 million grant in 2021. That same year, she also won a $450,000 Young Investigator Program grant from the U.S. Air Force and a $557,000 CAREER grant from the Nation- al Science Foundation to support her research.
Instead of applying an after-the-fact code to correct errors, Kamal’s approach relies on manipulating qubits with tiny pulses of microwave radiation that can keep them entangled.
“We’re not directly controlling the qubit itself, but you are controlling the environment around it and basically changing the way it behaves,” she says. Done the right way, such a system could become self-correcting: “It’s not the user, it’s the environment around the qubit that detects something is wrong and nudges the qubit in the right direction.”
As an analogy, imagine trying to get a ball into a hole during a game of miniature golf. Current error correction approaches are like a person aiming for the hole with a series of precise taps to correct its motion, while Kamal’s approach is more like designing the course with a wide trough that sends the ball into the hole no matter how the player hits it.
The Research Continues: Making the Mysteries Work for Us
Graduate student Emery Doucet ’22 compares it to a physics tool called Kapitza’s pendulum, which is able to balance a pencil on its point by applying a vibrating driving force. “If you are bored in class, you can spend as much time as you want trying to balance a pencil on its point, and it will never stand up right,” he says. “Using microwaves or lasers to turn the quantum system into a driven system is like taking that pencil and shaking it up and down really fast, so it can stand up despite all the little air currents and people walking by and cars shaking the building that make it fall.”

The experimental research, meanwhile, happens at the government’s NIST Boulder Laboratory in Colorado, as well as at Raytheon BBN Technologies in Cambridge, Massachusetts. There, graduate student Tristan Brown ’22 helps Kamal’s collaborator, experimental quantum physicist Leonardo Ranzani, apply the microwave drives to the qubit systems. “It’s very gratifying to make a prediction theoretically, and then see it play out in actual hardware,” says Brown.
The system consists of a blue metal barrel suspended upside down from the ceiling, in which the qubits are kept in their supercooled superconducting environment. That is connected through a tangle of multicolored wires to various electronic components that are able to apply the micro-waves through the housing. Already, the team has achieved some success with this approach. “We’ve been able to create an entangled state of two qubits and preserve it indefinitely,” Kamal says. “The question is if we can make this entanglement spread to 10 or 20 qubits to create really large, entangled states.”
Kamal is the ideal collaborator for the research, says Ranzani, given her background in both experimental and theoretical quantum physics. “She has a very good understanding of the theoretical, as you would expect, but also has the ability to understand where the limits are and what you can actually do in the lab,” he says.
If those limits are overcome and quantum computing delivers on its promise, then it will be due to the effort of Kamal and others, who have been able to wrestle the tiny but powerful invisible forces and make their mysteries work for us.